Myth: Lift is dependent on the shape of the wing
Reality: Lift is NOT dependent on the shape of the wing!
A key part of Bernoulli’s description of lift is that the shape of the wing is responsible for the development of lift. It requires that the wing have significant camber (curvature of the top of the wing). This is sometimes referred to as the “hump theory”. We guess that this conjured requirement is due to flailing attempts to explain why air flows faster over the top of the wing. However, in the Bernoulli description, there has been no satisfactory explanation for the acceleration of the air on the top of a wing making the description of lift incomplete at best.
If lift depended on the shape of the wing, only symmetrical wings could fly upside down. However, many airplanes have non-symmetrical wings and fly upside down just fine. Some people attempt to account for this by changing the physics of flight when inverted. Flight obeys the same physics to develop lift whether the aircraft is right side up or inverted. We will show that, in principle, any wing shape can fly inverted.
The reality of flight is the airfoil (cross-sectional shape) of the wing has nothing to do with lift, but it has a lot to do with efficiency at cruise speeds and stall characteristics.
Lift on a Wing
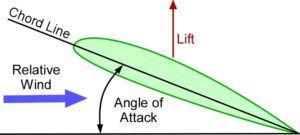
Angle of Attack
An engineer defines a wing’s angle of attack (AoA) as the angle between the wing’s direction of flight and the wing’s chord. The wing’s chord is a straight line between the leading edge and the trailing edge, as shown in Figure 1 We call this angle of attack the geometric angle of attack (gAoA) because it is defined by the details of the wing and not its performance in flight.
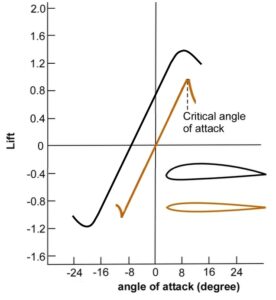
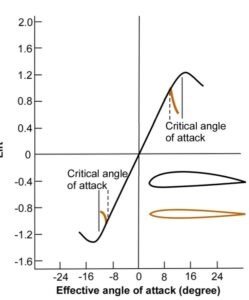
Figure 2 shows the lift for two very different wings as a function of both positive and negative geometric angles of attack. Lift is a straight line until the wing begins to stall. When one reads or hears about AoA it is always the gAoA.
Next, we will define a key concept: the effective angle of attack (eAoA): For any wing, one can find an angle into the wind where the wing has zero lift. This is true for the wing of a Boeing 747, a barn door, or holding your hand out of a moving vehicle’s window. The effective angle of attack (eAoA) is the angle measured from the wing’s orientation with zero lift.
Lift on the wing is exactly proportional to the eAoA. This is true for all wings, for both positive and negative angles. This is shown in Figure 3 for the same two wings as in Figure 2. (see Ref [2] for over 100 similar examples). When measured the same way, all wings fall on the same line. The slope is a straight line for all wings until the wing stalls.
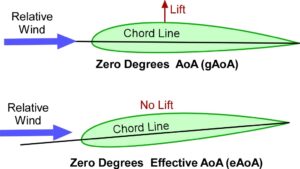
Effective Angle of Attack
It is important to emphasize that engineers define the angle of attack – the geometric AoA – in a different way that has nothing to do with the lift on a wing. You may have read or heard that a wing can have lift for a zero angle of attack. This is true for the fat, asymmetric wing in Figure 2. The difference is the result of the two different definitions of angle of attack.
Figure 4 shows the difference in wing orientation of a highly cambered wing for zero degrees gA0A and zero degrees eAoA.
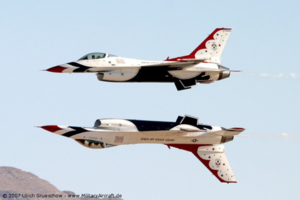
Referring to Figure 3, all wings have the same slope of lift versus eAoA but they differ in their stall characteristics. The sharp wing stalls at a smaller angle of attack and more abruptly than the blunt wing with camber.
The negative lift for negative angles of attack is just the performance of the wing inverted. Thus, we see why any wing shape can fly upside down. This is demonstrated nicely in Figure 5. Note that the wings are almost flat with almost no thickness.
Wing Airfoils
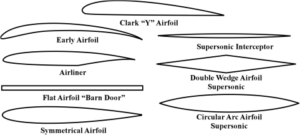
Figure 6 shows a variety of wing airfoils. The Clark “Y” airfoil is what we have been taught to think of a wing’s shape. But except for S.T.O.L. airplanes (Short TakeOff and Landing) and some experimental aircraft, almost all wings of airplanes since WWII have symmetrical or nearly symmetrical shapes. One example is the popular training aircraft – the Cessna 172. The difference in path length measured from the center of the leading edge to the wing’s trailing edge is only a few percent. So, the wing shape is asymmetrical but only to a small degree. The wing’s relatively blunt leading edge and slight camber (curved upper surface) provide good low-speed handling and stall characteristics that are excellent for a training aircraft.
Though all these wings have the same lift as a function of eAoA, when corrected for area and aspect ratio, they have very different cruise efficiency and stall characteristics. The Clark “Y” has good low-speed stall characteristics but would not be practical for supersonic flight. The Supersonic Interceptor wing is great for supersonic flight but its stall is so abrupt there is almost no warning.
The “early airfoil” in Figure 6 is shaped as it is because it was believed that a wing had to be thin to be efficient. The solution was to make a biplane with all the support structures external to the wing and therefore exposed to the wind. The Wright Brothers did good work with their wind tunnel, but they never put a support wire in the wind tunnel to test for the drag produced. If they had, the Wright Flyer may have looked a little different. A good example of a wing similar to the early airfoil can be seen in Figure 7, which is a photo of the Tern in Flight.
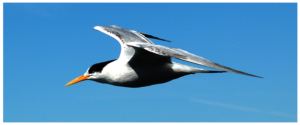
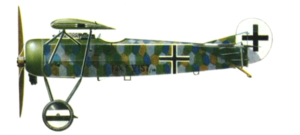
The first example of a Clark “Y” type wing was the German WWI Fokker D VIII shown in Figure 8. It was a monoplane, and unlike the biplanes, most of the structure was inside the wing. It was developed at the end of WW I and was a deadly fighter. But it came too late in the war to make a significant impact. The Fokker D VIII was effective because it flew slower than any other airplane without stalling thereby providing excellent handling and maneuverability. It had this capability because of the fat, cambered wing. This forced an attacker to fly past it and become a target. Thus, the hunter became the prey. It was the only weapon that was turned over to the Allies in the Treaty of Versailles.
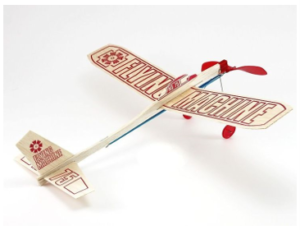
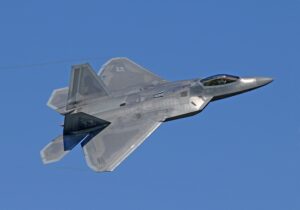
One of the best examples of an airfoil that goes counter to the teaching of lift being dependent on the shape of the wing is the Flat Airfoil (a.k.a. the barn door). Examples of this wing design are the Thunderbirds in Figure 5, the toy airplane in Figure 9, and the F-22 in Figure 10. The wing on the toy airplane is not that different from that of the Supersonic Interceptor’s wing.
Take Away
- A wing’s airfoil (shape of the wing) has nothing to do with lift, but only cruise efficiency and stall characteristics.
- For all wings, the lift is proportional to the effective angle of attack.
- Any wing can be flown inverted in principle.
- Any description of lift that depends on the shape of the wing is wrong.
Now that we have stated the shape of the wing is not important to the production of lift, we can go on to another misconception of flight. That is the motion of the air over the wing is basically horizontal.
References
1. David F. Anderson and Scott Eberhardt. Understanding Flight, 2nd ed. (McGraw-Hill, 2010).
2. Ira H Abbott and Albert E. Eberhardt, Doenhoff, Theory of Wing Sections (Dover Publishing, Inc., 1959).